In the current global context, increasing energy demand and fluctuating fuel prices have heightened the importance of energy savings [1]. The quest for efficiency in the use of energy resources has become imperative to address direct and indirect energy consumption in various human activities [2]. According to [3], the lack of thermal efficiency in buildings can result in daily energy consumption that quickly surpasses the energy used in the manufacturing of construction materials. In recent years, the evolution of construction methods and materials has responded to the growth of ideas such as sustainability, energy efficiency and environmental respect [4]. This paradigm shift has permeated society and led to a re-evaluation of how architecture, along with its materials, leads the transition towards sustainability. However, access to these new materials, mostly minerals and synthetics, is limited to countries with resources for integration into national building codes.
The construction sector, responsible for approximately 32% of global final energy use and 36% of greenhouse gas emissions [5], exhibits significant variations between countries due to social, economic, energy requirements and focus on energy codes. In this context, trend projections for 2050 suggest an increase in the proportion of energy consumption in the building sector [6]. In response to these challenges, the concept of passive house is introduced as a construction standard aimed at reducing energy consumption in residential buildings [7]. The authors in [3] note that this approach seeks to improve thermal comfort for occupants while maintaining low energy consumption, using natural resources to achieve efficiency, either passively or through technologies adapted to the needs of the inhabitants.
In the context of thermal comfort, studies have been conducted in regions with climates like that of the Colombian Caribbean, such as Brazil, India, Indonesia, and Australia. These studies investigate strategies to mitigate heat and humidity, revealing the effectiveness of both passive and active strategies to improve thermal comfort. In Brazil, the studies align with ASHRAE 55 standards [8], [9]; in India, there is an emphasis on natural ventilation [10]; in Indonesia, there is a high dependence on air conditioning [11]; and in Australia, the importance of the habits of occupants is highlighted [12]. These approaches are essential for developing sustainable solutions in the Caribbean.
Despite these advances, the author in [13] highlights the scant attention to thermal and energy quality in Social Interest Housing (SIH) in Latin America. The lack of attention to these variables in residential construction, especially in the Latin American context, underscores the need for effective and affordable strategies to improve energy efficiency and the quality of life for residents. The implementation of low thermal demand housing, aimed at achieving sustainable development, becomes a requirement imposed on various global economies.
In Colombia, regulations concerning thermal comfort and energy efficiency in buildings, particularly in SIH, are still evolving and face significant limitations. Despite the presence of international standards such as ASHRAE 55, EN 15251, and ISO 7730, these standards do not fully cater to the specific climatic conditions of the country, underscoring the necessity for dedicated local studies [14]. The Colombian Technical Standard NTC 5316, which closely mirrors the ASHRAE 55 standard, is recommended but not mandatory for building design. Furthermore, Resolution 5926 of 2011 from the District Environment Secretariat and Resolution 0549 of 2015 from the Ministry of Housing have introduced policies aimed at eco-efficient buildings and the obligatory reduction of water and energy consumption in new housing. However, these resolutions lack detailed guidelines to ensure adequate thermal comfort [15].
In this context, recent research by [16] highlights the importance of adapting evaluation methodologies and tools to Colombian conditions. Moreover, other studies address the urgent need to update regulations and standards to ensure sufficient thermal comfort in various climatic zones of the country [17]. Researchers in [18] emphasises the lack of consideration for basic constraints such as climatic zone or orientation in the construction of most homes, resulting in low thermal comfort, indoor pollution and health problems for residents. A promising solution to this issue in SIH is the adoption of passive climate control, adapted to the local climate, offering thermal comfort and air quality with low consumption of non-renewable energy sources [19]. This solution is based on architectural design that incorporates passive solar heating and cooling, using local and modern materials to maintain effective construction costs and reduce environmental impact.
The characterization of the local climate at any geographical point is a fundamental factor in the design of residential constructions [20]. This climatic influence lays the foundation for decision-making on bioclimatic strategies, whether passive or active [21], [22], to maintain a good sense of thermal comfort in indoor environments [23]. The lack of consideration for these studies can lead to adverse impacts on health due to thermal discomfort, and another influenced aspect is the quality of the indoor environment [24]. In this scenario, the availability of climatic data and energy assessment software, such as Climate Consultant 6.0 used to determine passive climate control systems [25], and Energy Plus to check the thermal performance of different strategies employed for energy demand reduction [26], facilitates the response to the needs of bioclimatic constructions [27], [28].
As a result, a crucial initial step is to capitalise on site advantages, considering solar orientation and the local meteorological microclimate. On-site diagnosis helps identify local wind characteristics that will influence the microclimate. Conversely, good housing orientation becomes the most important factor in efficiently harnessing passive solar heat gain or loss, considering architectural attributes [29]. These features include south, north and side-oriented window areas, roof slope, architectural roofs, or overhangs that provide solar protection at noon, as well as the impact of trees in the adjacent or nearby area to the dwelling [30].
This study addresses the relationship between climatic behaviour, thermal comfort and energy performance in SIH. The results arise from field research conducted in 120 housing units inspected in 8 municipalities in the Departamento del Magdalena located in the Colombian Caribbean region. This fieldwork included the measurement and observation of the structural conditions of the houses, allowing for a precise characterization of the construction elements and occupancy conditions. Given that climatic behaviour in the 8 municipalities is uniform concerning thermal comfort and other climatological variables associated with the microclimates of each of these areas, as demonstrated in this research, a case study was performed characterizing all variables that influence the thermal comfort of residential constructions, focusing on SIH located in the study municipality (Plato Magdalena). For such an analysis, an average house was defined based on data collected from 120 bioclimatic units located in the study areas. The climatological data, sourced from meteorological stations whose geographical locations are detailed in Table 1, were analysed for the period spanning from 2020 to 2022. EnergyPlus and Climate Consultant tools were implemented to verify the impact of climate, construction elements and use on internal conditions, using the auto-adaptive comfort model. Our study pioneers an innovative approach to understanding the interaction between climatic behaviour, thermal comfort and energy efficiency in social interest housing constructed in Colombia.
The climatological variables are presented in a region characterised by the intertropical convergence zone, with a bimodal rainfall pattern. The study encompasses 8 municipalities in the Colombian Caribbean region of the Departamento del Magdalena (Table 1), which, according to the climate classification Caldas -Lang, fall under a warm arid and semi-arid climate [31]. However, it also exhibits characteristics of a warm semi-humid climate, as illustrated in Figure 1.
Geographic location of meteorological stations in the study municipalities
Municipality | Latitude [°N]/Longitude [°E] |
---|---|
Plato | 9.857049 -74.651558 |
Chibolo | 10.005528/ -74.610627 |
Sabanas de San Ángel | 10.058423/ -74.209294 |
Santa Bárbara de Pinto | 9.436145/ -74.708228 |
Nueva Granada | 9.661306/ -74.32692 |
Guamal | 9.275781/ -74.059988 |
Zona Bananera | 10.800478/ -74.14337 |
Sitio Nuevo | 11.005854/ -74.683676 |
Climatic classification in the study region
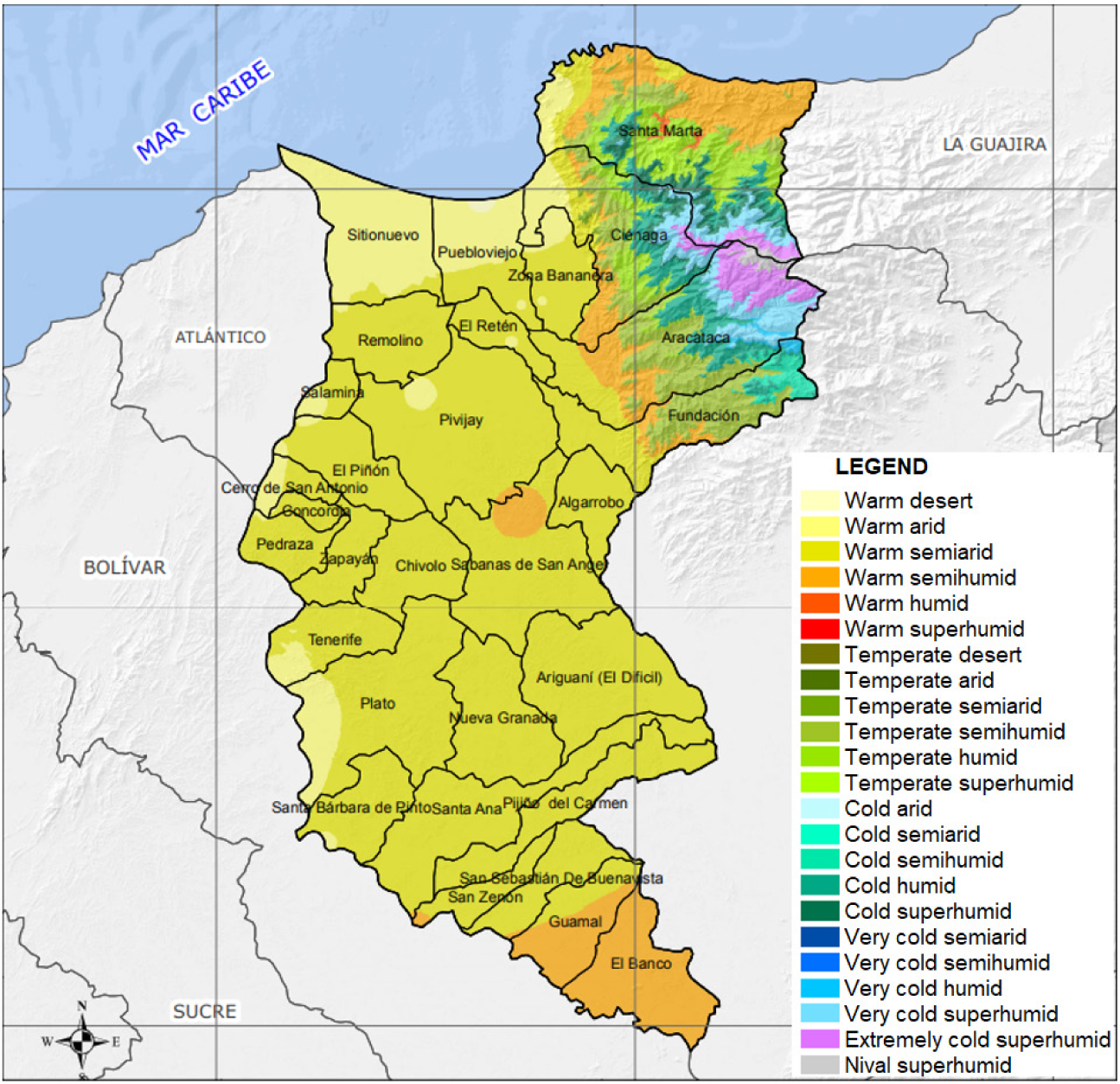
With the purpose of examining the variables that impact the thermal comfort of residential buildings in each of these areas, a first approach related to thermal sensation (TS) is the comfort index (CI) of the human body, conditioned to meteorological variables such as ambient temperature or dry bulb temperature (td), relative humidity (φ) and wind speed (w), which were adjusted for use in Colombia, particularly in warm and hot zones [32]. When the air temperature exceeds 25 °C with a relative humidity of 80%, thermal discomfort (TD) occurs, causing a sensation of discomfort and high perspiration, raising body temperature between 38.9 °C to 40 °C. The air loses the capacity to absorb vapour, resulting in the accumulation of heat in the human body.
Eq. (1) and Table 2, provided by [33], are adjusted for the CI at elevations lower than 1000 m above sea level:
(1)
Classification of experienced sensation
CI | TS |
---|---|
0 to 3 | Very Hot |
3.1 to 5 | Hot |
5.1 to 7 | Warm |
7.1 to 11 | Pleasant |
11.1 to 13 | Somewhat Cold |
13.1 to 15 | Cold |
Over 15 | Very Cold |
To estimate how temperature, humidity and wind affect the thermoregulatory capacity in relation to a person's thermal comfort sensation, the Missenard index was proposed [34], known as net effective temperature (tNET). This index is widely used in literature related to meteorological topics and is defined by eq. (2):
(2)
To determine the thermal discomfort temperature tTD in the study areas, empirical eq. (3) was used, as provided by Landsberg for tropical climates [35]:
(3)
where td represents the dry bulb temperature (°C), and tw denotes the wet bulb temperature (°C).
In this regard, according to the authors in [36], the thermal comfort range lies between 21 °C and 25 °C of effective temperature, with a relative humidity below 80%. Thermal discomfort begins between 25 °C and 26 °C of effective temperature for individuals residing in hot zones, and between 21 °C and 22 °C for those unaccustomed to such conditions.
For the identification of the local thermal environment based on temperature and humidity comfort criteria, nine environmental conditions are adopted, as depicted in Figure 2, based on the combination of temperature and humidity comfort thresholds provided in [37], [38]. An annual average operating temperature is used to define the comfort range. Temperatures below the lower acceptability limit of the adaptive thermal comfort range (tc,l) are identified as the cold condition, while temperatures above the upper acceptability limit of the adaptive thermal comfort range (tc, u) are considered the hot condition.
Psychrometric chart, identification of local thermal environment based on temperature and humidity comfort criteria
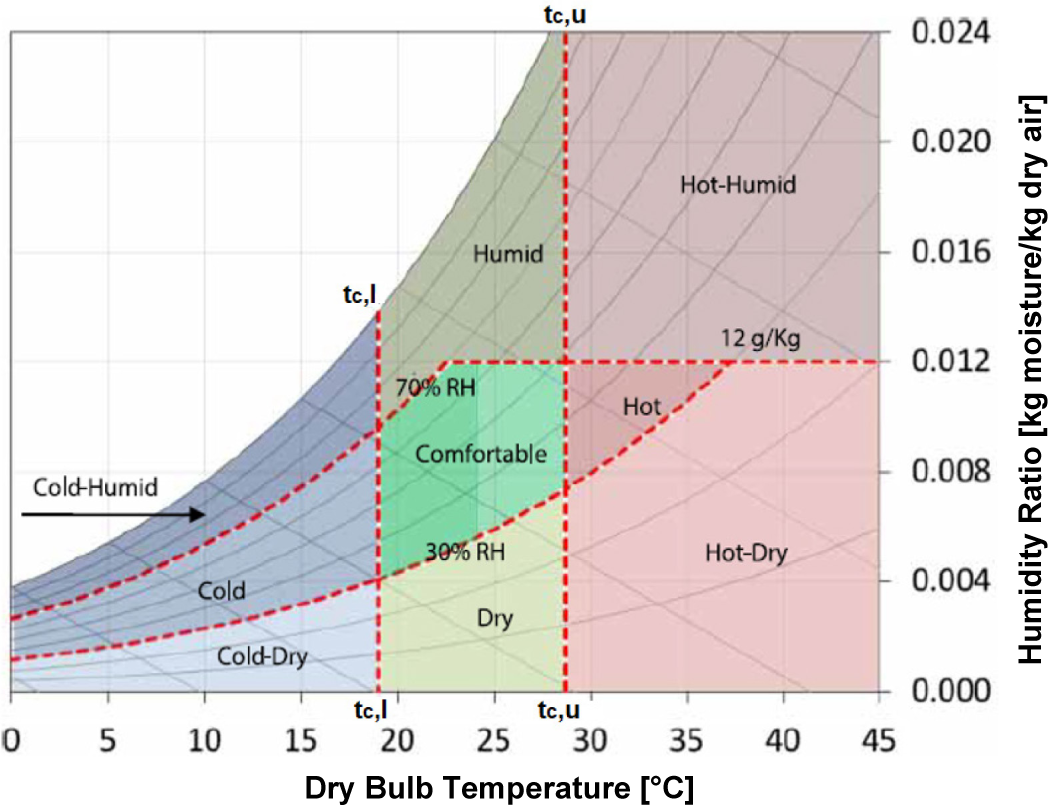
Comfort criteria for humidity are defined by both relative humidity and absolute humidity content in the air. The humidity comfort zone is considered between 30% and 70% of φ and less than 12 g/kg. Humidity exceeding 70% φ or higher than 12 g/kg is considered a humid condition, and less than 30% φ is deemed a dry condition. An intersection of temperature and humidity comfort conditions is defined as a comfortable environment. Another combination of temperature and humidity criteria defines the remaining eight environmental conditions, namely cold-humid, cold, cold-dry, humid, dry, hot-humid, hot and hot-dry.
Regarding the case study, the analysed climatological data were taken from the municipality in the central-southern part of the Departamento del Magdalena, on the eastern bank of the Magdalena River, which forms its boundary with the Departamento de Bolívar. Its climate, classified as warm arid, features daily temperatures ranging from 21 °C to 35 °C, with consistency throughout the year; the hottest months are April and August, according to Figure 3. In terms of thermal comfort, referred to as operative temperature, the adaptability limits established by the ASHRAE 55-2010 model, using natural ventilation, range from 23.4 °C to 29.3 °C. It is observed that during the hottest months, the average stands at 28.7 °C, close to the maximum thermal comfort limit.
Temperature range and comfort zone limits for the municipality under study
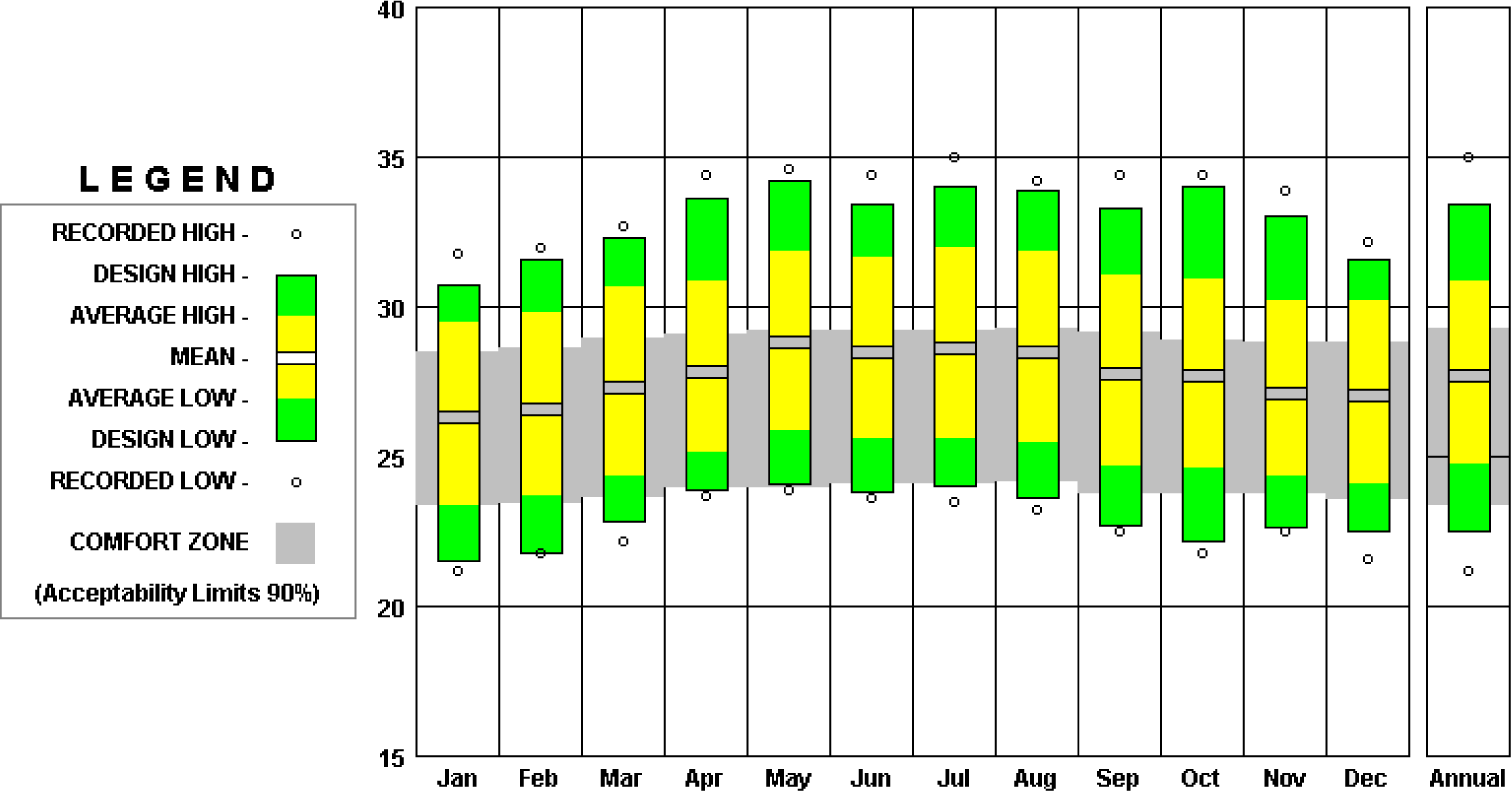
Concerning td and tw, which are determinants for φ, the daily td is maintained in a range of 21.2 °C to 35 °C. The tw fluctuates between 19.6 °C and 29.9 °C (Figure 4a and Figure 4b); when these temperatures are close, the φ is high, indicating that the air contains a significant amount of water vapor in relation to its maximum capacity at that temperature. It is established that the annual average percentage values of φ reach a maximum of 96.7% and a minimum of 67.8% (Figure 4c), meaning that the air is fully saturated with water vapor, impacting the thermal comfort of the inhabitants in the area. This high level of humidity, combined with high temperatures, creates a sensation of humid heat.
a) Dry bulb temperature; b) Wet bulb temperature; c) Daily relative humidity
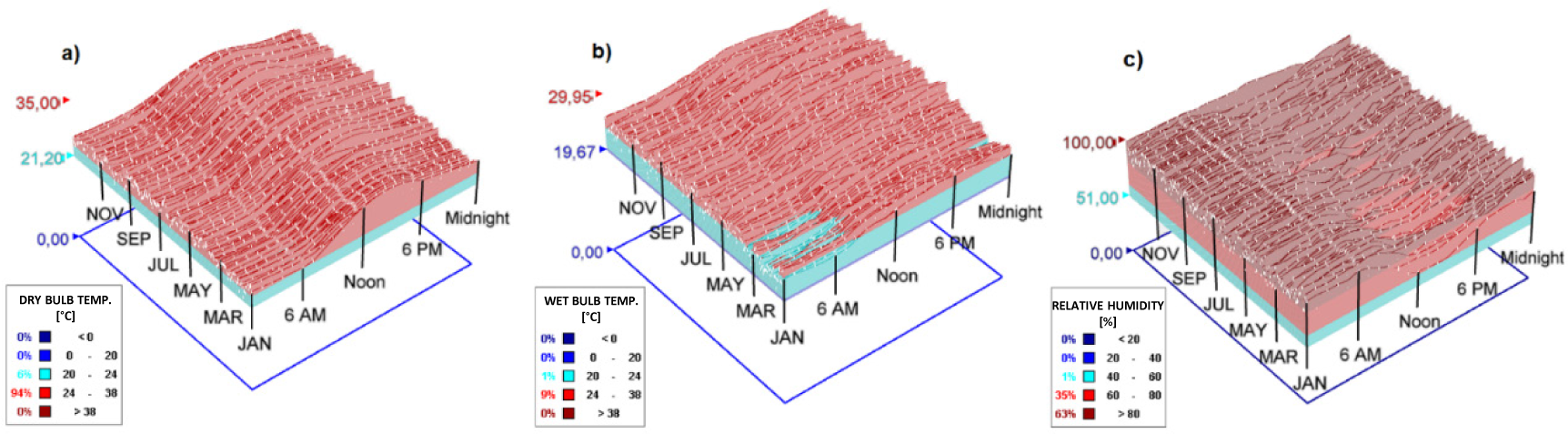
Figure 5 provides a monthly analysis of the behaviour of radiation variables in the study area, contrasting theoretical values with data measured by meteorological stations. It is emphasised that solar radiation reaches daily peaks in March and September, recording 1121 Wh/m2, with a maximum average value of 850 Wh/m2 in March and 775 Wh/m2 in September. In contrast, the lowest values range between 600 Wh/m2 and 640 Wh/m2 from October to December, coinciding with the months of highest precipitation according to Figure 6. This precipitation pattern directly impacts the duration of usable sunlight hours, crucial data for the analysis of the implementation of active solar technologies such as photovoltaic systems. The precipitation pattern in this area is bimodal, with two dry seasons, one more pronounced from December to April and another in the middle of the year. Rainy seasons occur in each semester, with the second, from September to November, being wetter than the first.
Daily average radiation (global, direct, diffuse) for the municipality under study
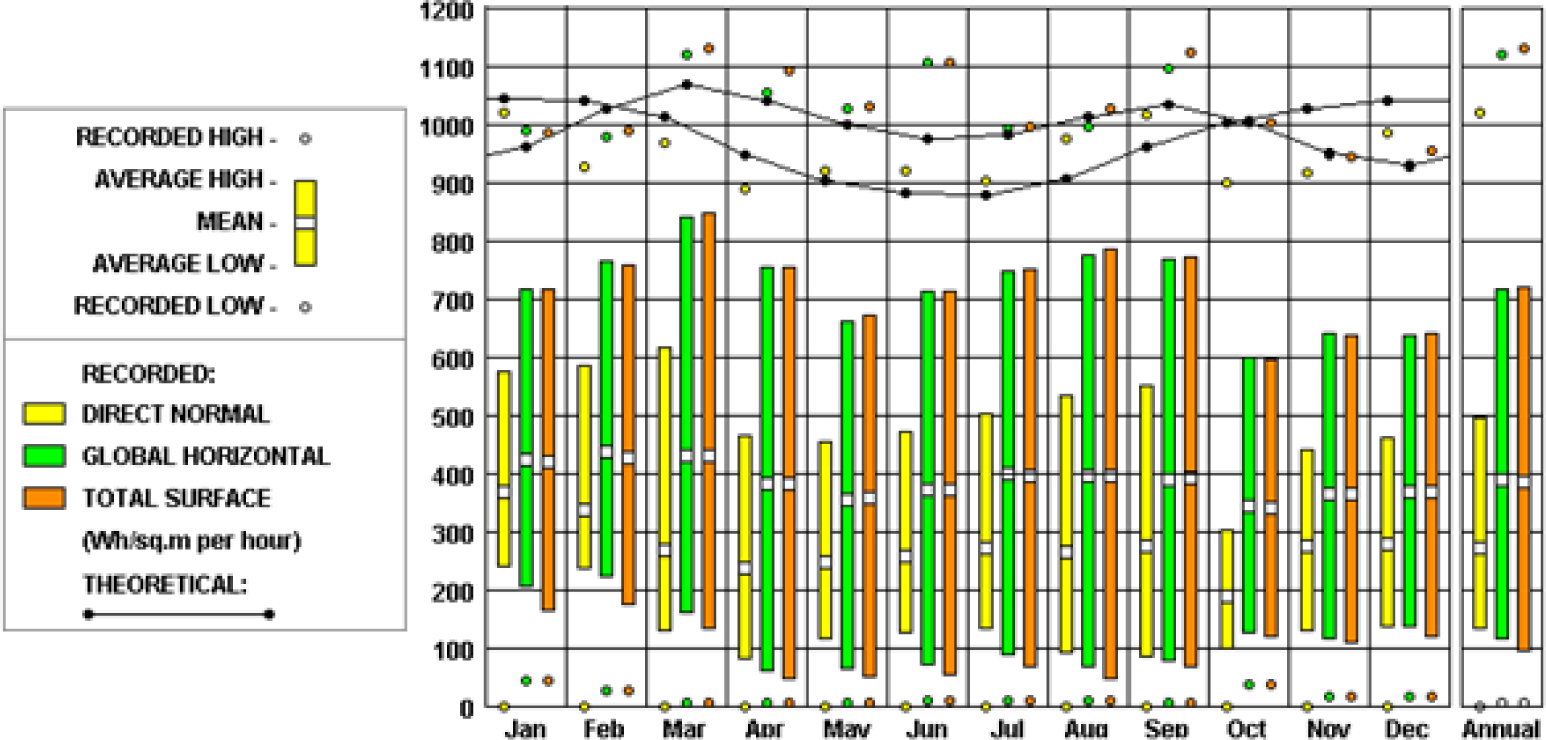
Monthly average precipitation for the municipality under study
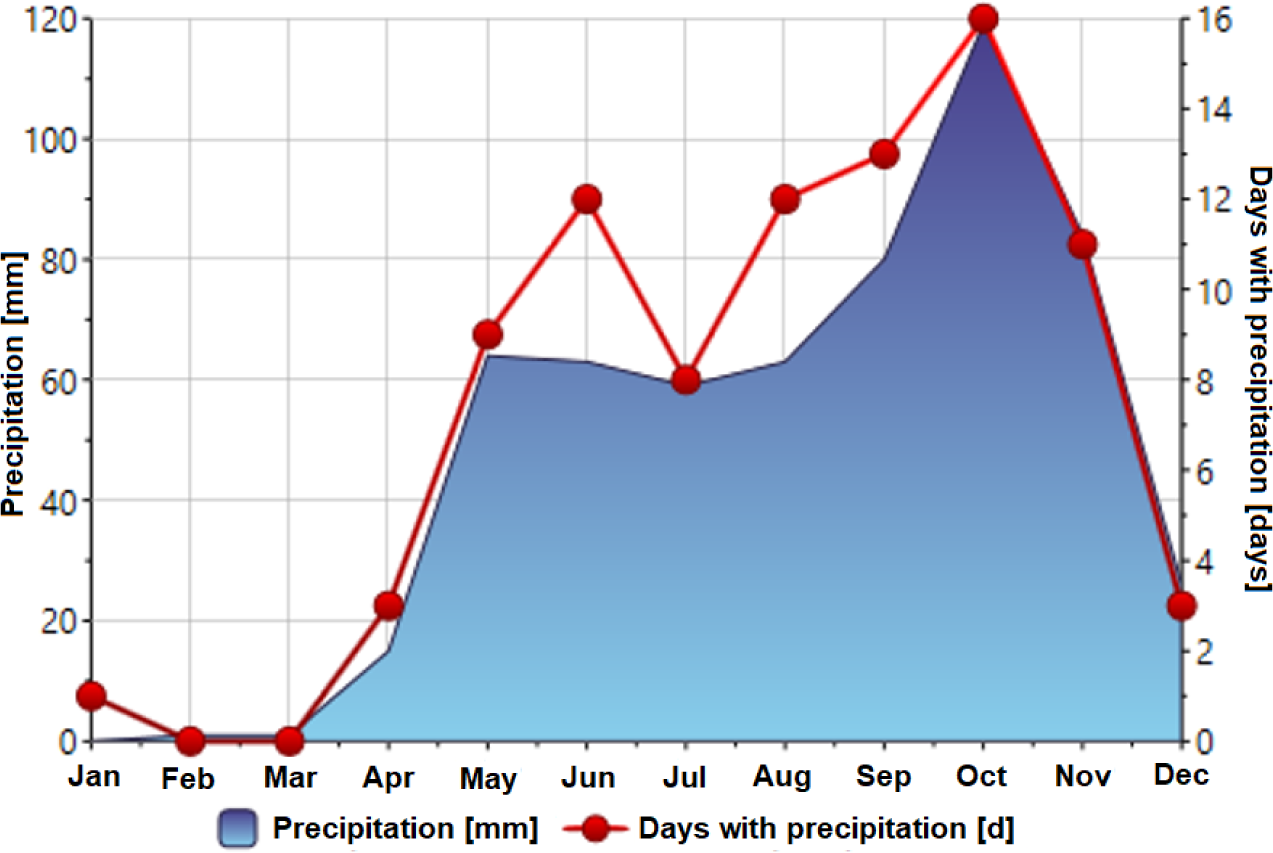
Figure 7 details the characteristics of wind speed, both monthly and daily, with a maximum average monthly peak of 3 to 5 m/s and a range of 50%. In the daily behaviour, maximum speeds of 15.3 m/s are observed with a low range of 2%. An essential variable for passive climatization strategies is natural ventilation, linked to wind speed and direction in the study area. The wind rose reveals that the predominant direction varies between northeast and southeast, with maximum average monthly speeds of 3.9 m/s in February and 1.6 m/s in October, with air temperatures between 24 °C and 27.7 °C and relative humidity above 70%.
Annual wind rose for the municipality under study
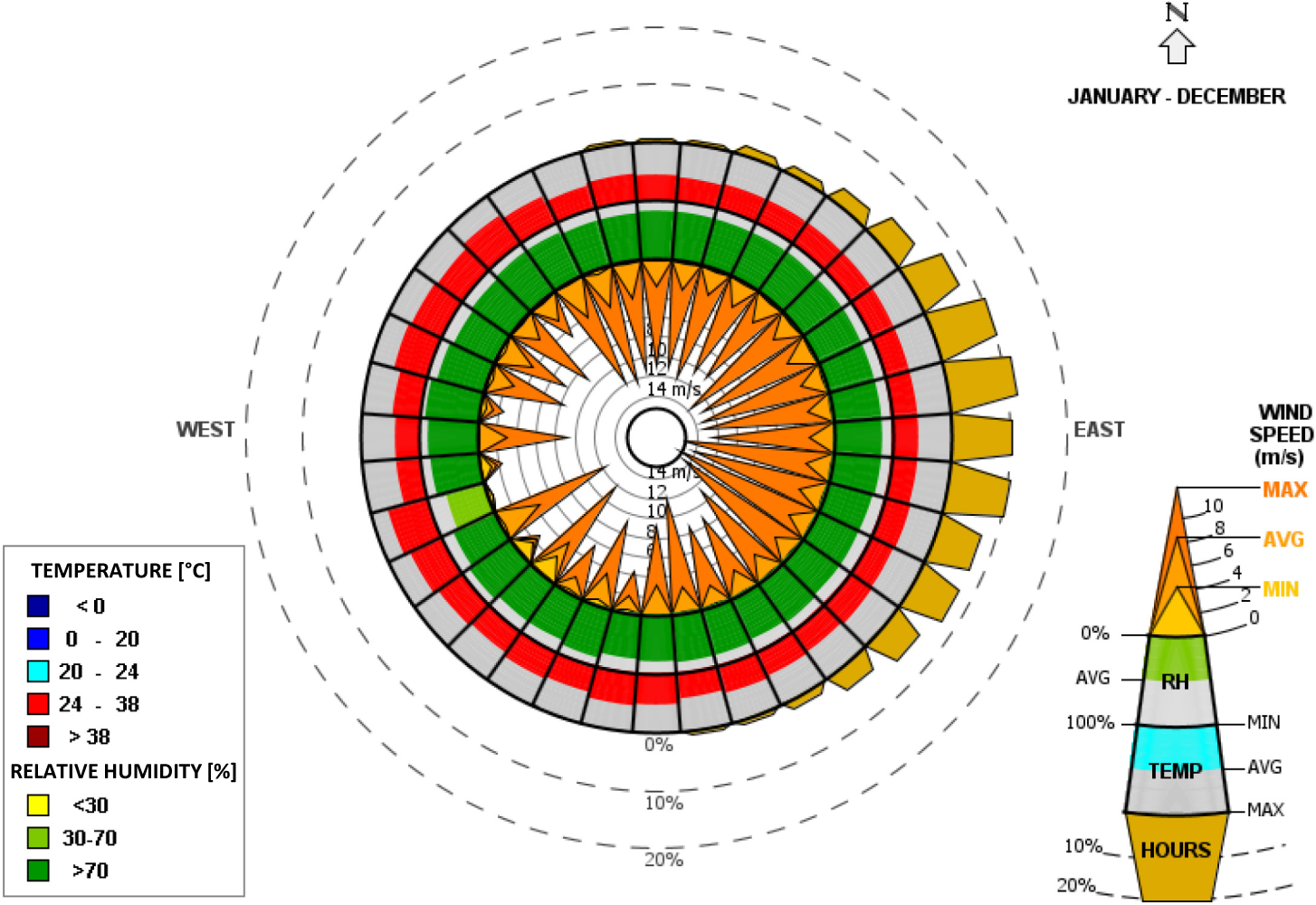
Regarding the characteristics of the SIH implemented in the Departamento del Magdalena, an average built area of approximately 60 m2 is observed, distributed across three thermal zones comprising a living room, kitchen and two bedrooms. The structure of these houses can be visualized in Figure 8a, while their internal zoning is detailed in Figure 8b.
Typical SIH in the Departamento del Magdalena (left); b) Internal distribution of zones (right)
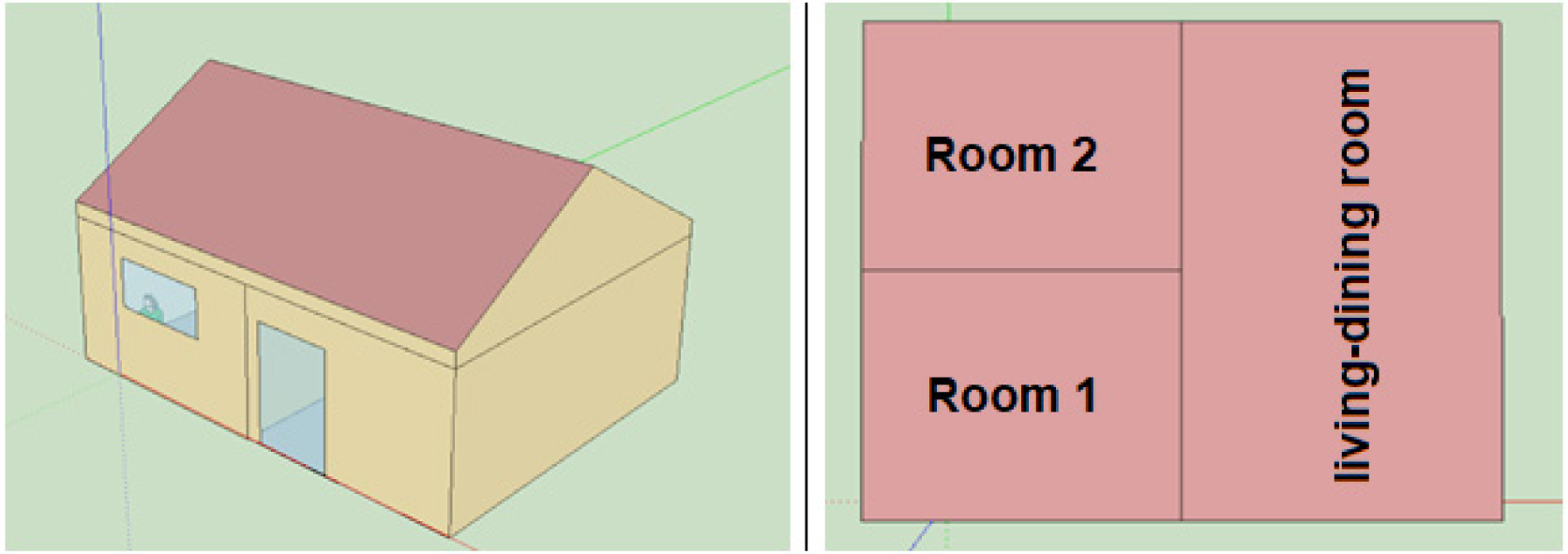
Concerning the construction characteristics of the building envelopes and internal loads of occupancy and lighting, they are presented in Table 3. In this table, the typical transmittance of the houses is at U=2.39 W/m2K, resulting in high heat transfer and elevated thermal inertia that does not favour achieving optimal comfort conditions, especially given the high climatic severity conditions prevailing throughout the region.
To verify comfort in the dwelling of the generated scenario, the operative temperature was determined for each of the three thermal zones: bedroom 1, bedroom 2 and the living-dining room. This was done with the purpose of applying the adaptive comfort model, which establishes suitable ranges considering interactions throughout a typical year of climate and all dynamic flows generated by usage and construction components. Characteristics derived from dynamic simulation through EnergyPlus as a detailed analysis tool include a base model representing a typical SIH with construction trends used in the department, a model with insulation improving envelope quality by adding 5 cm of material to reduce heat transmission, a model with cross-ventilation to quantify the impact of utilising winds, and a model with shading combining the previous improvements, considering the use of external shading or ventilated facades to mitigate severe climatic conditions.
Typical characteristics of SIH in the Municipalities of Departamento del Magdalena
Data | Characteristics |
---|---|
Location | 9.857049°N/ -74.651558°E |
Building Type | SIH |
Area per Floor | 63 m2 |
Number of Thermal Zones | 3 |
% Glazing vs. Wall | 6.7% |
External Walls | Stucco 0.025; 200 mm Brick; Plaster 0.019 (Transmittance U=2.39 W/m2K) |
Glass Type | Single 6mm Glass (Transmittance U=2.39 W/m2K) |
Internal Load for Lighting | 12 W/m2 |
Internal Load per Person | 4 persons |
Internal Load for Electrical Equipment | 120 W/person |
The outcome in Table 4 regarding the experienced thermal sensation in the 8 municipalities is warm. It is observed that there is no climatic variability in the study areas. The effective temperature for a good thermal sensation should average 23.4 °C. Discomfort sets in from 25.6 °C onwards. Wind speeds exhibit a behaviour of gentle breezes on average at 3 m/s according to the Beaufort scale [39]. Relative humidity maintains a high and constant average of 80%. In this case, to dissipate metabolic heat, specialised literature suggests thermoregulation resources [40], such as forced ventilation (fans) and cooling systems (air conditioning) [41]. Other natural mechanisms, such as natural ventilation [42], and physiological transpiration, which regulates heat exchange in the body, help balance body temperature.
Effects of temperature, humidity and wind speed in 8 municipalities of Departamento del Magdalena
Municipality | Elev. [masl] | td [°C] | tw [°C] | φ [%] | w [m/s] | tNET [°C] | tTD [°C] | CI | TS |
---|---|---|---|---|---|---|---|---|---|
Plato | 10 | 27.8 | 24.3 | 82 | 2.5 | 23.8 | 25.6 | 3.8 | Hot |
Chibolo | 133 | 27.7 | 24.1 | 81 | 2.8 | 23.5 | 25.5 | 3.9 | Hot |
Sabanas de San Ángel | 129 | 27.8 | 24 | 80 | 2.7 | 23.6 | 25.5 | 3.8 | Hot |
Santa Bárbara de Pinto | 19 | 27.7 | 24 | 80 | 2.9 | 23.4 | 25.5 | 3.9 | Hot |
Nueva Granada | 114 | 27.7 | 24 | 80 | 3.1 | 23.3 | 25.5 | 3.9 | Hot |
Guamal | 36 | 27.8 | 24.3 | 81 | 2.5 | 23.8 | 25.6 | 3.8 | Hot |
Zona Bananera | 37 | 27.7 | 24.3 | 81 | 4.0 | 22.9 | 25.6 | 4.0 | Hot |
Sitio Nuevo | 4 | 27.8 | 24.2 | 81 | 3.7 | 23.2 | 25.6 | 3.9 | Hot |
Figure 9 presents the hourly traceability of temperature and humidity data throughout the year in the study area, represented on the psychrometric chart. The climatic condition covered by the data ranges between cold-humid, humid and hot-humid. In this context, the ASHRAE 55-2010 adaptive comfort model was evaluated with natural ventilation, allowing occupants to open and close windows, and its thermal response partly depends on the external climate [43]. This model assumes that occupants adjust their clothing to thermal conditions and adopt a sedentary behaviour. It is observed that, out of the 8760 hours in the year, approximately 5137 hours provide thermal comfort, representing 59% of the time, while the remaining 41% of hours do not offer a comfortable sensation within the buildings.
Psychrometric chart for adaptive comfort in the studied municipality
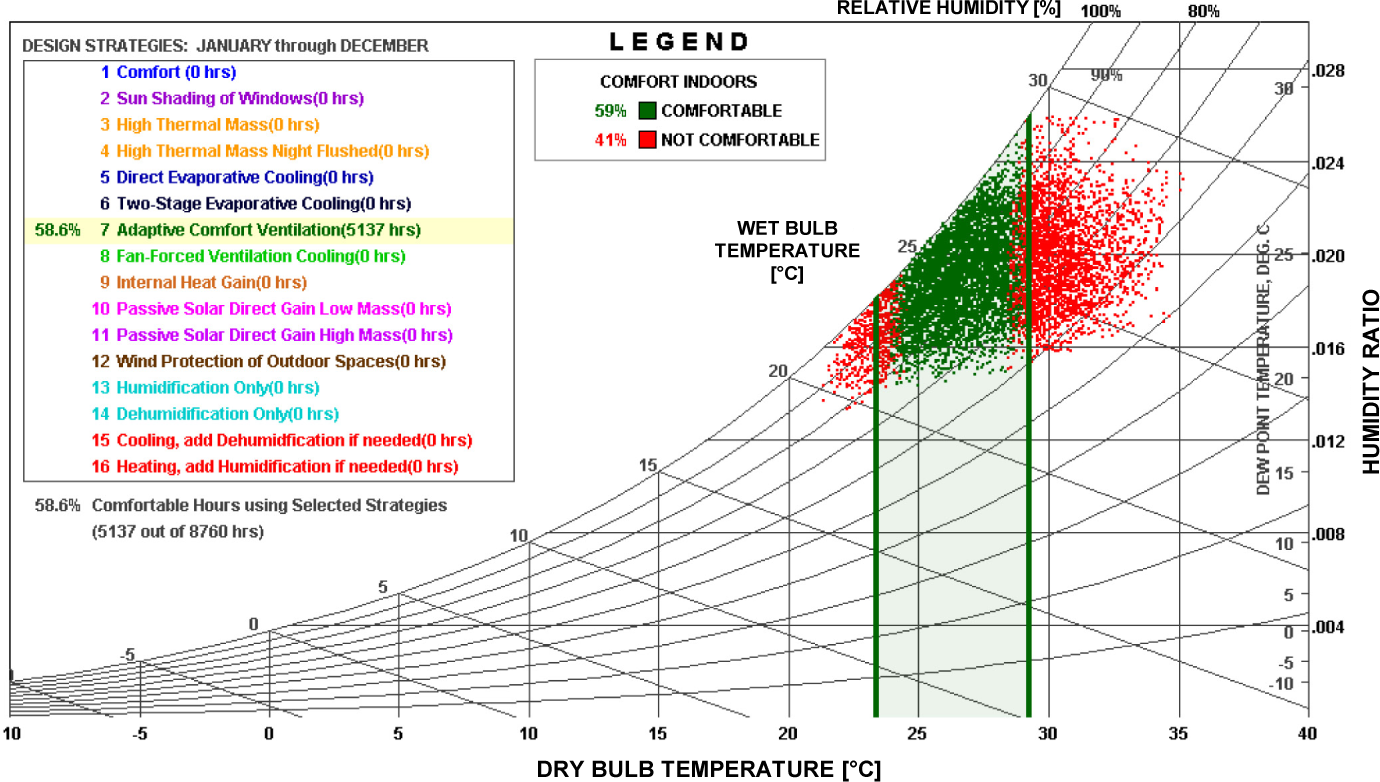
In the study area, characterised by high temperatures and relative humidity, implementing strategies for residential air conditioning and dehumidification is imperative, as shown in Figure 10. This is crucial to achieve 100% thermal comfort throughout the 8760 hours of the year. The comfort model proposed is based on the California Energy Code, 2019 [44], which indicates that, to properly size residential air conditioning systems, indoor dry bulb design conditions should range between 20 °C and 23.9 °C. Although the code does not specify humidity limits, an 80% relative humidity and 18.9 °C wet bulb are used for the upper limit, and -2.8 °C dew point for the lower limit. The proposed design strategies include implementing solar protection on windows (27%, equivalent to 2362 hours), using adaptive comfort with natural ventilation (58.6% comfort, i.e., 5137 hours), forced ventilation for cooling (5.7% comfort, 503 hours), dehumidification (5.7%, 503 hours) and cooling (35.2%, 3082 hours).
Psychrometric comfort chart for the municipality under study, covering 100% of the year
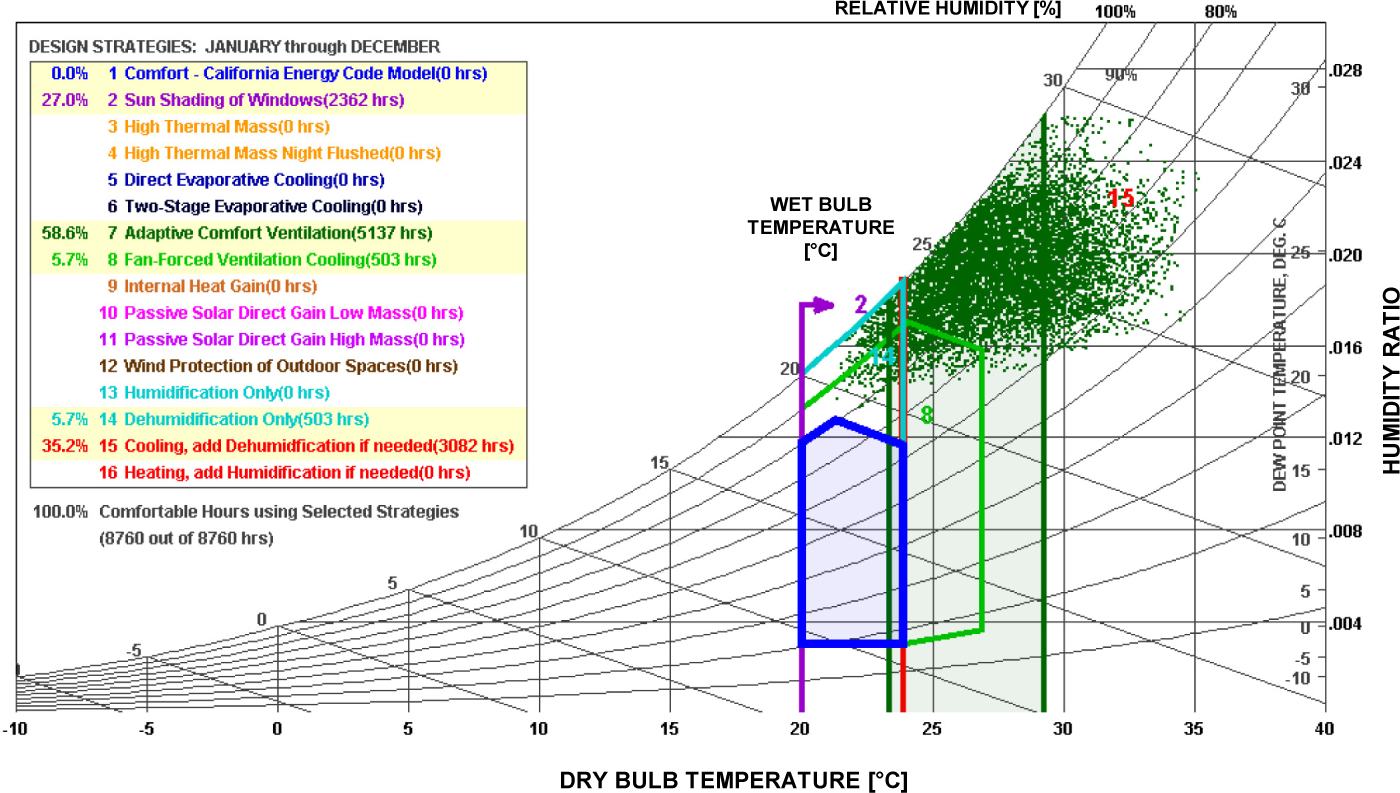
In a context more closely linked to the socioeconomic conditions of the studied population, which belongs to a lower social stratum, strategies can be implemented to slightly improve the thermal comfort sensation in the SIH (Figure 11). Three design strategies are proposed: solar protection on windows, achieving 27% comfort for 2362 hours; adaptive comfort through natural ventilation, reaching 58.6% comfort for 5137 hours; and forced ventilation for cooling, with 5.7% comfort for 503 hours. The total annual comfort percentage is established at 61.3%, equivalent to 5372 hours per year.
Psychrometric chart for solar protection on windows, forced ventilation and adaptive comfort
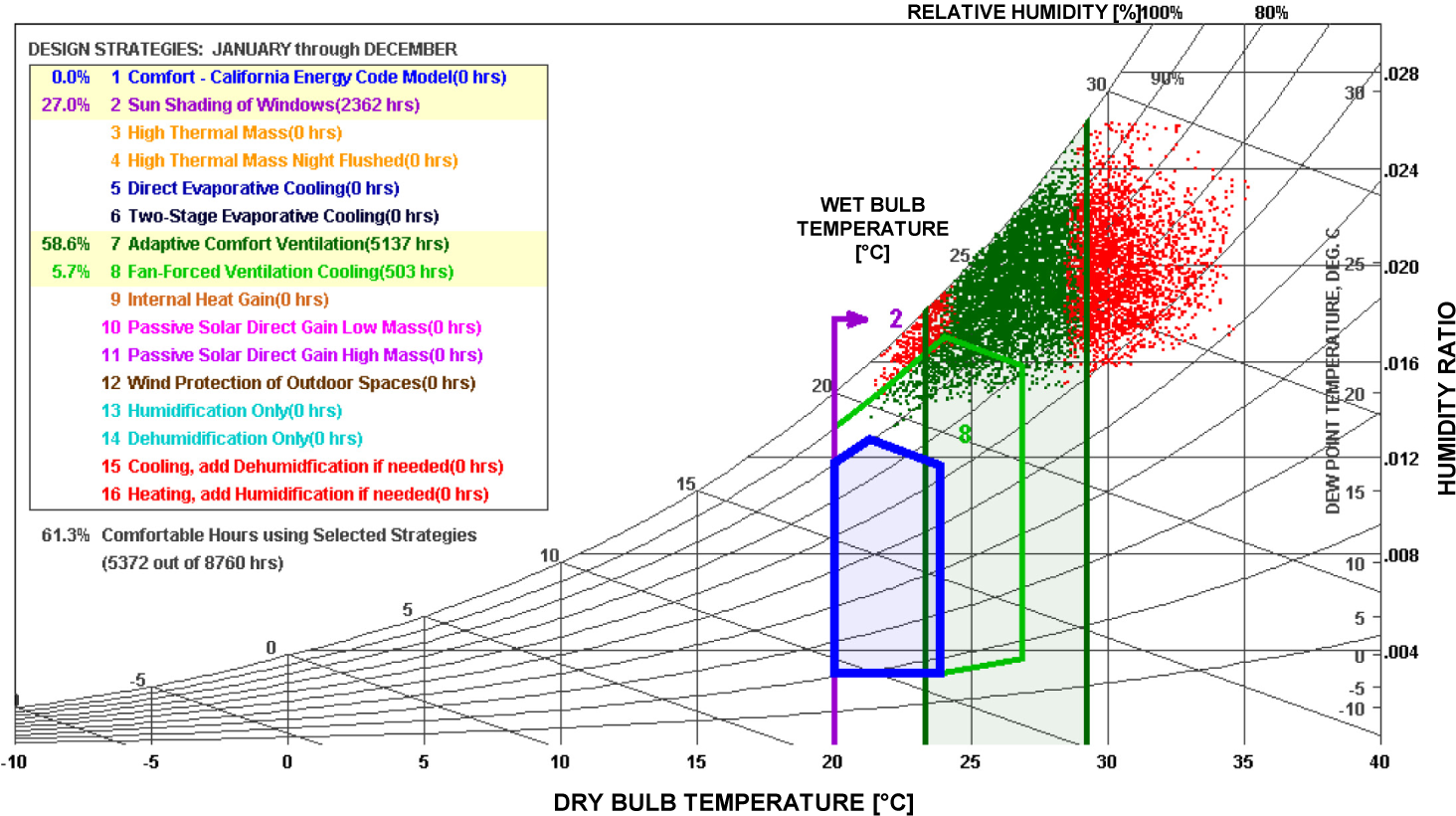
Next, the thermal performance of different strategies used for reducing energy demand in the study municipality is presented, considering the base model. In Figure 12a, the maximum and minimum operative temperatures recorded in room 1 are observed, reaching values of 47.5 °C and 25.8 °C, respectively, with a variation range of approximately 21.4 °C for these extremes over a typical year. Regarding the average operative temperature during the same period, it is 33.2 °C, surpassing the established limits for thermal comfort.
In relation to the operative temperature of room 2, Figure 12b illustrates extreme data for a typical year, ranging between 45.4 °C and 25.3 °C. The maximum temperature is slightly lower than room 1, approximately 2 °C less, owing to the orientation of the area, which receives less direct radiation and experiences mitigated effects from the thermal inertia of the materials. Similarly, the thermal zone designated as the living-dining room exhibits extreme historical records between 48.7 °C and 25.3 °C, as depicted in Figure 12c. The maximum operative temperature surpasses the two previously analysed thermal zones due to the large external surfaces exposed to direct solar radiation and extreme temperatures.
Finally, the roof has a gable configuration that reduces direct exposure to the sun during certain hours of the day. Composed of asbestos or asbestos cement sheets, a highly carcinogenic and harmful material, this roof has considerable thermal inertia that affects comfort conditions, especially in a severe climate like that of Departamento del Magdalena. The operative temperatures in this area are more extreme, with maximum and minimum records of 56.4 °C and 23.1 °C, respectively, as shown in Figure 12d. The impact of the improvements implemented can be evident, encompassing the three fundamental strategies aimed at reducing the internal thermal demand of the zones, thus improving comfort conditions and, consequently, strengthening resilience to climate change.
Operative temperature base model: a) room 1; b) room 2; c) living-dining room; d) roof
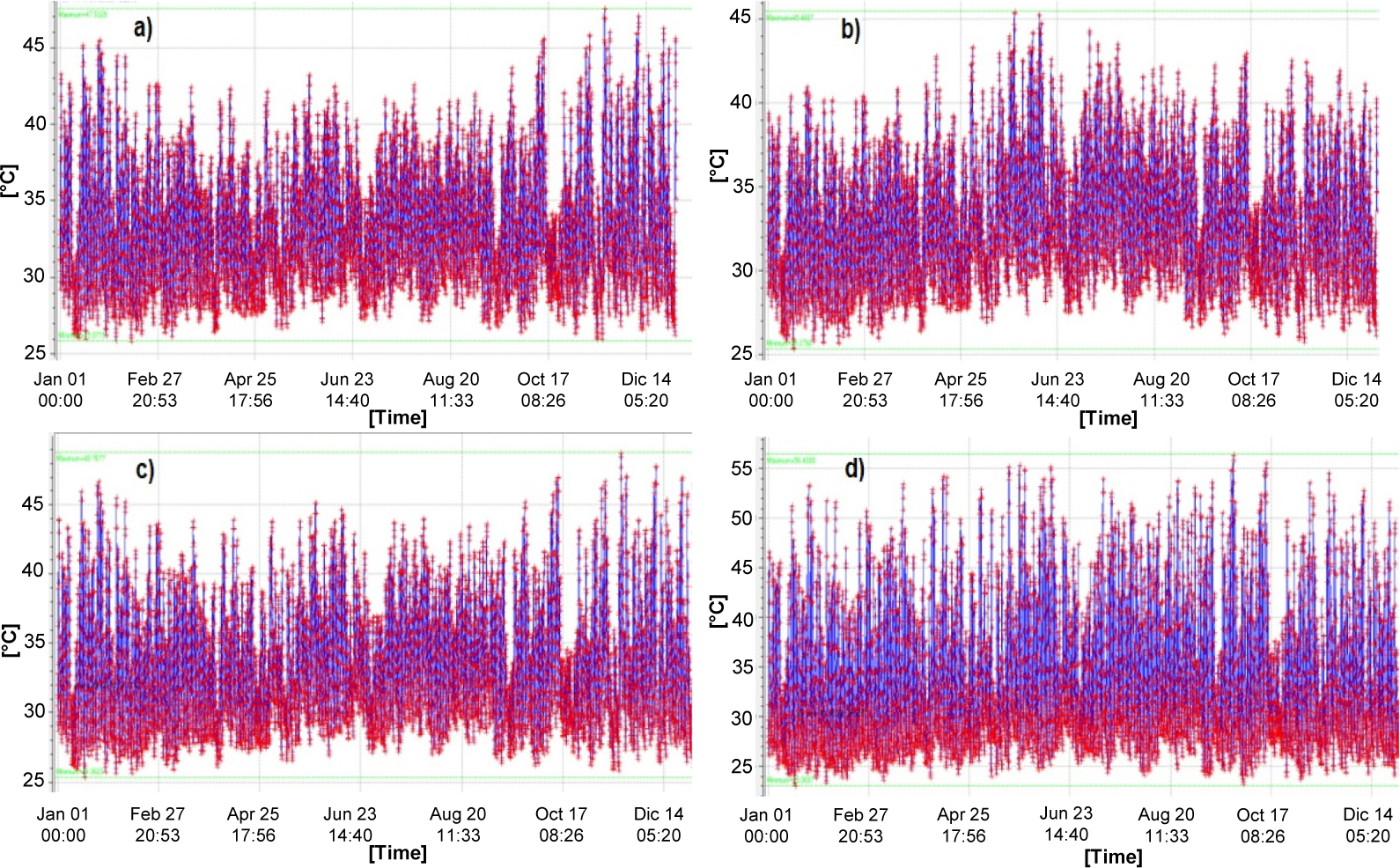
To better understand the thermal behaviour across different areas of the house throughout the year, Figure 13 presents the monthly averages of maximum, mean, and minimum temperatures for Bedroom 1, Bedroom 2, Living-dining room, and Roof. These averages help illustrate the patterns observed in Figure 12, providing a clearer picture of how temperature fluctuates over time and highlighting the importance of insulation, shading, and ventilation strategies to optimise thermal comfort and energy efficiency.
Monthly averages of temperatures in different areas of the house
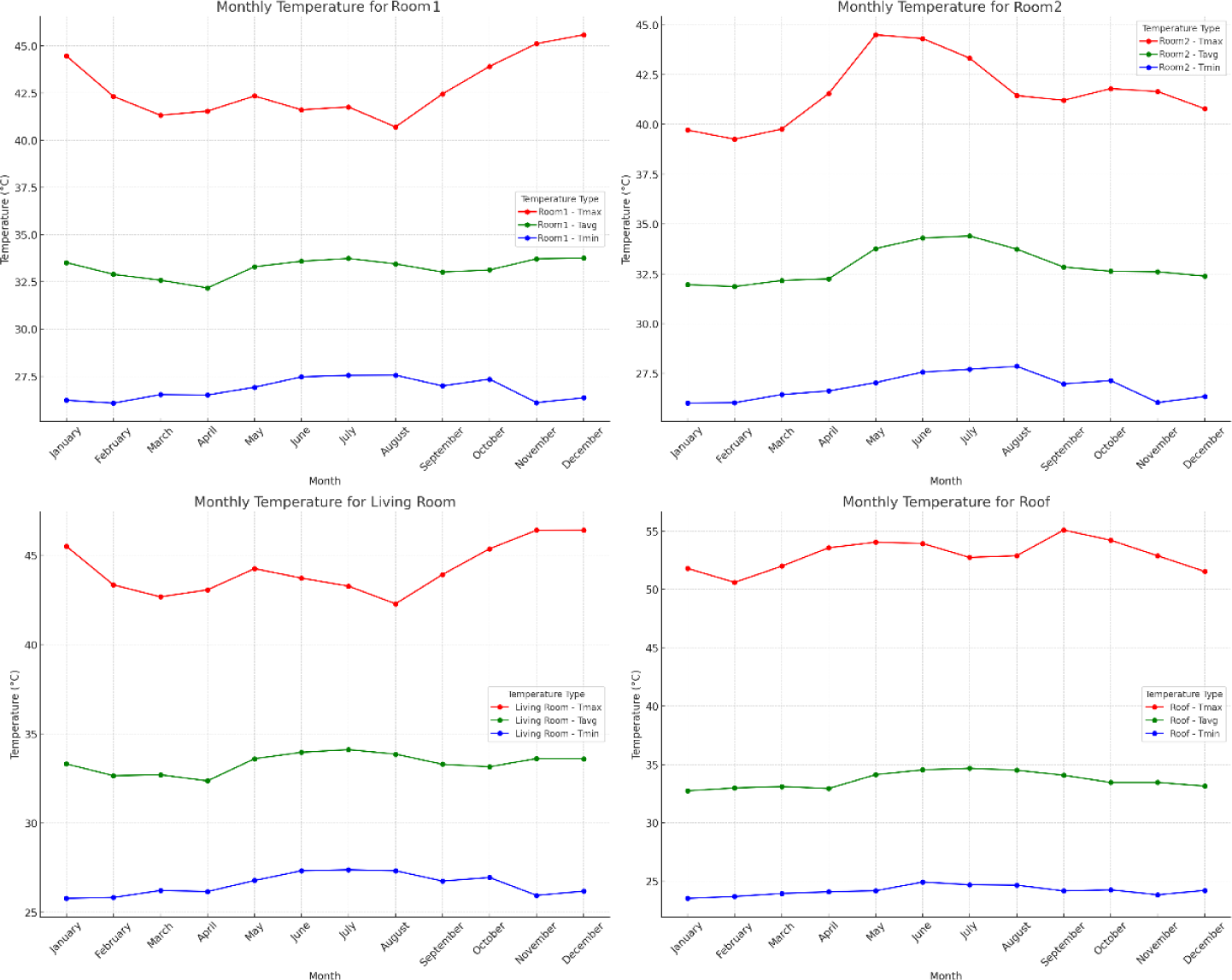
The envelope improvement strategy includes the addition of 5 cm of insulation, achieving a significant reduction in transmittance, as shown in Figure 14, with a decrease of 12 °C below the maximum operating temperature in all thermal zones compared to the base model (Figure 12). This reduction is achieved by reducing the heat flow through the envelope, highlighting the importance of exterior insulation, in accordance with regulations and construction codes aimed at mitigating thermal demand in homes and buildings, thereby reducing energy impact. Additionally, there is a reduction in the storage capacity of the envelope, which manages to maintain lower temperatures for certain periods during the year (February – October) for bedroom 1 and the dining room.
Behaviour of the SIH with envelope improvement: a) bedroom 1, b) bedroom 2, c) living-dining room; d) roof
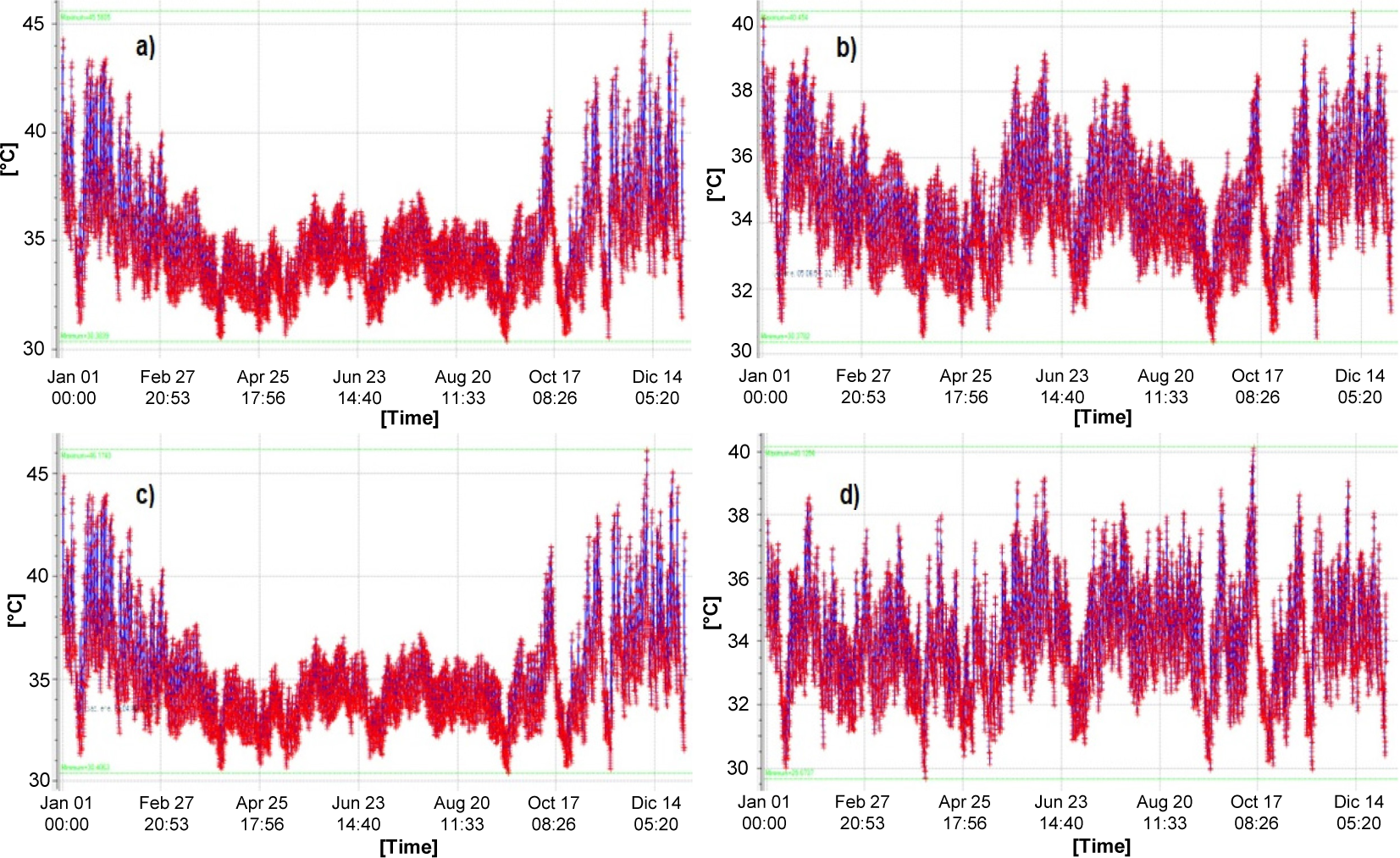
In Figure 15, insulation and cross-ventilation through openings were considered, achieving a significant reduction in the maximum operative temperature compared to the base model, approximately 15 °C in each of the zones. However, peaks are observed for certain periods of time when the external temperature is severe. This is because the air circulation occurs at temperatures higher than the comfort zone. Heat accumulates more significantly in the roof, as illustrated in Figure 14d to Figure 16d, due to several factors: direct solar radiation, the thermal inertia of fibre cement, convection of warm air, and thermal mass.
Behaviour of the SIH with cross-ventilation and envelope: a) bedroom 1; b) bedroom 2; c) living-dining room; d) roof
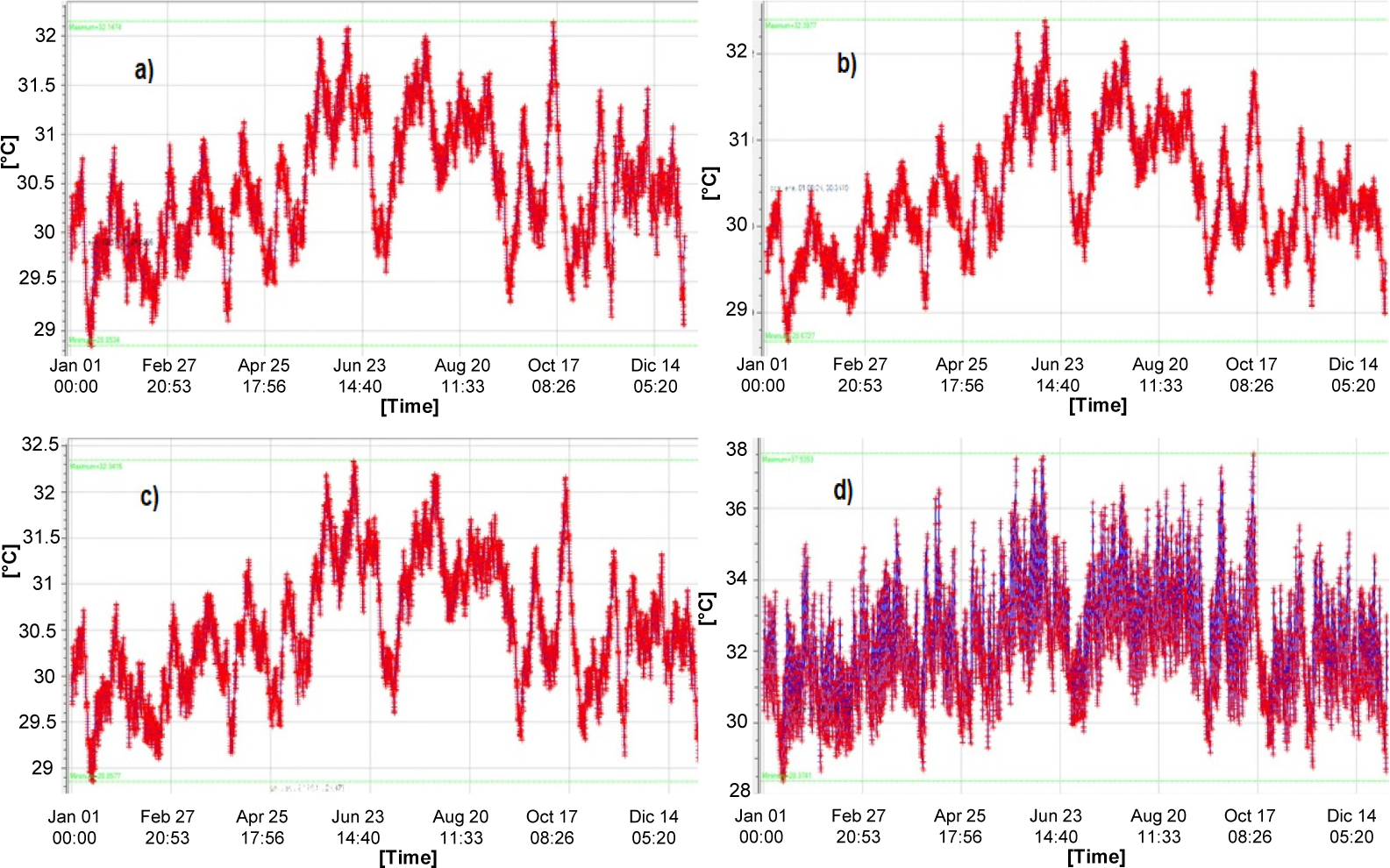
Direct radiation has a significant impact on comfort conditions in the Departamento del Magdalena, emphasising the importance of reducing it to improve the indoor environment in each dwelling. To achieve this saving, shading on the east and west sides was considered, resulting in the most significant reductions in the dwelling's operative temperatures. These decreases are approximately 17 °C below the operative temperature of the base model (Figure 15). Therefore, the implementation of wall shading systems and the use of ventilated facades emerge as crucial solutions to cope with the severe climate in the Departamento del Magdalena.
Performance of the SIH with shading: a) bedroom 1; b) bedroom 2; c) living-dining room; d) roof
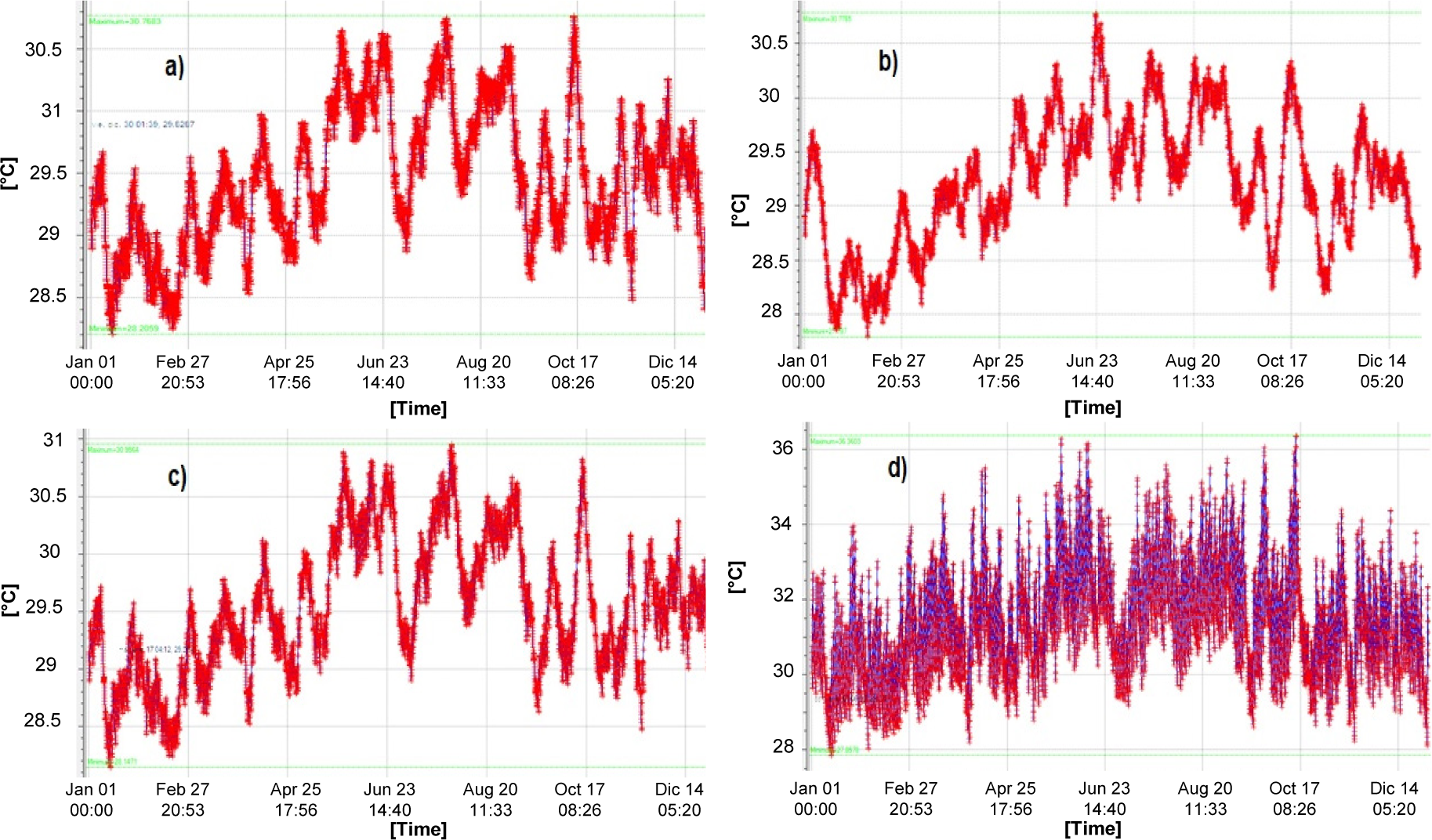
Research on climate conditions and housing characteristics in Departamento del Magdalena reveals an arid warm climate with constant daily temperatures ranging between 21 °C and 35 °C. The adaptive comfort model is recommended for designing air conditioning and dehumidification strategies, crucial in a high-severity climate, achieving a 61.3% annual comfort rate. Results highlight areas of thermal discomfort and construction challenges, especially the need to reduce direct solar gain.
Improvement proposals include reducing transmittance through insulation use, coupled with cross-ventilation and shading, demonstrating their effectiveness in lowering operative temperatures. The climatic uniformity in analysed municipalities translates to hot thermal conditions, with operative temperatures exceeding 24.0 °C, surpassing the comfort threshold set at 23.4 °C. Constant high relative humidity at 80% and gentle breezes with wind speeds of 2.5 to 4.0 m/s suggest challenges for metabolic heat dissipation, posing conditions conducive to thermal discomfort.
Thermoregulation strategies such as forced ventilation and air conditioning, along with natural mechanisms for balancing body temperature, are recommended. In the socio-economic context, specific improvements are proposed for lower strata, achieving significant reductions in operative temperatures through the use of insulation in the housing envelope, cross-flow of air, and shading. The envelope improvement strategy underscores the importance of exterior insulation, while shading, especially in east and west areas, shows the most significant reductions. These strategies not only enhance thermal comfort but also strengthen resilience to climate change in the Departamento del Magdalena, providing valuable insights for sustainable and efficient architectural design in the area. For the proposed strategies, the integration of renewable energy systems, such as photovoltaics, is recommended to harness the high solar radiation in the area. This approach would enhance the energy efficiency of social housing, decrease reliance on non-renewable energy sources, and promote the environmental and economic sustainability of local communities.
The diverse climatic zones of Colombia, ranging from tropical forests to mountainous regions, present unique challenges and opportunities for thermal comfort and energy performance in SIH. The methodologies and tools employed in this study are adaptable to various climatic contexts, facilitating simulations and analyses that consider local climate variables. By conducting similar inspections and simulations across these diverse climates, findings can validate the general applicability of identified strategies. This methodological approach can contribute to identifying best practices in construction, which may subsequently inform building codes or standards tailored to each climatic zone in Colombia.
The authors appreciate the support of the research groups of the Universidad de la Costa and the Universidad del Magdalena in the project “Investigación de los efectos de la variabilidad climática y el cambio climático sobre el recurso hídrico, biodiversidad y actividades agropecuarias en el Departamento del Magdalena”
td | dry bulb temperature | [°C] |
tNET | net effective temperature | [°C] |
tTD | thermal discomfort temperature | [°C] |
tw | wet bulb temperature | [°C] |
U | transmittance | [W/m2K] |
w | wind speed | [m/s] |
φ | relative humidity | [%] |
SIH | Social Interest Housing |
TS | Thermal Sensation |
CI | Comfort Index |
Building energy-consumption status worldwide and the state-of-the-art technologies for zero-energy buildings during the past decade ,Energy Build , Vol. 128 ,pp 198–213 , 2016, https://doi.org/https://doi.org/10.1016/j.enbuild.2016.06.089
, Sustainable development: Meaning, history, principles, pillars, and implications for human action: Literature review ,Cogent Soc Sci , Vol. 5 (1), 2019, https://doi.org/https://doi.org/10.1080/23311886.2019.1653531
, Importancia de la construcción eficiente en la Patagonia. Universidad Nacional del Comahue ,Desde La Patagonia , Vol. 11 (18),pp 38–45 , 20142024
, Sustainable Building Standards, Codes and Certification Systems: The Status Quo and Future Directions in Saudi Arabia ,Sustainability , Vol. 14 (16),pp 10314 , 2022, https://doi.org/https://doi.org/10.3390/su141610314
, Passive cooling of buildings with phase change materials using whole-building energy simulation tools: A review ,Renewable and Sustainable Energy Reviews , Vol. 80 ,pp 1239–1255 , 2017, https://doi.org/https://doi.org/10.1016/j.rser.2017.05.139
, - , Financing the energy renovation of buildings with Cohesion Policy funding, 20142024
Design and realisation of the Passive House concept in different climate zones ,Energy Effic , Vol. 13 (8),pp 1561–1604 , 2020, https://doi.org/https://doi.org/10.1007/s12053-019-09819-6
, Thermal preference and comfort assessment in air-conditioned and naturally-ventilated university classrooms under hot and humid conditions in Brazil ,Energy Build , Vol. 211 ,pp 109783 , 2020, https://doi.org/https://doi.org/10.1016/j.enbuild.2020.109783
, - , Application of the adaptive model proposed by ASHRAE 55 in the Brazilian climate context: raising some issues, 2014
Investigation on window opening behavior in naturally ventilated hostels of warm and humid climate ,Energy Build , Vol. 268 ,pp 112184 , 2022, https://doi.org/https://doi.org/10.1016/j.enbuild.2022.112184
, Predicting Comfort Temperature in Indonesia, an Initial Step to Reduce Cooling Energy Consumption ,Buildings , Vol. 5 (3),pp 802–813 , 2015, https://doi.org/https://doi.org/10.3390/buildings5030802
, Window/door opening-mediated bedroom ventilation and its impact on sleep quality of healthy, young adults ,Indoor Air , Vol. 28 (2),pp 339–351 , 2018, https://doi.org/https://doi.org/10.1111/ina.12435
, - , Manual de diseño para viviendas con climatización pasiva, Alemania, 2002
The Importance of Standardised Data-Collection Methods in the Improvement of Thermal Comfort Assessment Models for Developing Countries in the Tropics ,Sustainability , Vol. 11 (15),pp 4180 , 2019, https://doi.org/https://doi.org/10.3390/su11154180
, Estimation of the impact of energy efficiency measures recommended by Colombian legislation on the performance of low- and middle-income housing buildings ,Journal of Building Engineering , Vol. 52 ,pp 104402 , 2022, https://doi.org/https://doi.org/10.1016/j.jobe.2022.104402
, Scoping Review of Thermal Comfort Research in Colombia ,Buildings , Vol. 11 (6),pp 232 , 2021, https://doi.org/https://doi.org/10.3390/buildings11060232
, Dynamic evaluation of thermal comfort scenarios in a Colombian large-scale social housing project ,Engineering, Construction and Architectural Management , Vol. 29 (5),pp 1909–1930 , 2022, https://doi.org/https://doi.org/10.1108/ECAM-09-2020-0684
, - , Metodología para evaluar la calidad del aire interior en diseño de VIS de clima frío, Tesis de Maestría, Universidad Nacional de Colombia, Bogotá, 2018
Optimization of the passive house concept for residential buildings in the South-Brazilian region ,Energy Build , Vol. 240 ,pp 110871 , 2021, https://doi.org/https://doi.org/10.1016/j.enbuild.2021.110871
, Computational Approach to Predict Thermal Comfort Levels at Summer Peak Conditions in Passive House Based on Natural Ventilation ,Journal of Sustainable Development of Energy, Water and Environment Systems , Vol. 10 (4),pp 1–21 , 2022, https://doi.org/https://doi.org/10.13044/j.sdewes.d10.0419
, Energy sufficiency in buildings, a synonym for passive and low energy architecture (PLEA) ,Archit Sci Rev , Vol. 61 (5),pp 292–297 , 2018, https://doi.org/https://doi.org/10.1080/00038628.2018.1505332
, Experimental Study and Analysis of Thermal Comfort in a University Campus Building in Tropical Climate ,Sustainability , Vol. 12 (21),pp 8886 , 2020, https://doi.org/https://doi.org/10.3390/su12218886
, Estudio del confort climático a escala micro-local. El caso de la ciudad de Bahía Blanca (Argentina) ,Bitácora Urbano Territorial , Vol. 27 (3),pp 91–100 , 2017, https://doi.org/https://doi.org/10.15446/bitacora.v27n3.56995
, Analysis of Thermal and Humidity Sensations in Educational Buildings in Eastern European Climate Conditions ,Journal of Sustainable Development of Energy, Water and Environment Systems , Vol. 11 (4),pp 1–17 , 2023, https://doi.org/https://doi.org/10.13044/j.sdewes.d11.0461
, Adaptation of climate-responsive building design strategies and resilience to climate change in the hot/arid region of Khartoum, Sudan ,Sustain Cities Soc , Vol. 47 ,pp 101429 , 2019, https://doi.org/https://doi.org/10.1016/j.scs.2019.101429
, - , Impact of solar radiation on building envelope using EnergyPlus Software, 7th International Mechanical Engineering Congress (SIMEC-2017), 2017
Development and validation of an HVAC on/off controller in EnergyPlus for energy simulation of residential and small commercial buildings ,Energy Build , Vol. 183 ,pp 467–483 , 2019, https://doi.org/https://doi.org/10.1016/j.enbuild.2018.11.005
, Influence of Input Climatic Data on Simulations of Annual Energy Needs of a Building: EnergyPlus and WRF Modeling for a Case Study in Rome (Italy) ,Energies (Basel) , Vol. 11 (10),pp 2835 , 2018, https://doi.org/https://doi.org/10.3390/en11102835
, Design of solar systems for buildings and use of BIM tools: Overview of relevant geometric aspects ,FME Transactions , Vol. 47 (2),pp 387–397 , 2019, https://doi.org/https://doi.org/10.5937/fmet1902387D
, - , Architectural Science and the Sun, 20182018
- , Climatological zoning according to the Caldas-Lang model of the Negro River basin using the geographic information system GIS”, (In Spanish, Zonificación climatológica según el modelo Caldas - Lang de la cuenca del río Negro mediante el uso del sistema de información geográfica SIG”), Specialization Thesis, Universidad Militar Nueva Granada, Bogotá, 2014
- , Calculations and strategies to mitigate the lack of thermal comfort in urban housing in warm humid climates (In Spanish, Cálculos y estrategias para mitigar la falta de confort térmico en la vivienda urbana del clima cálido húmedo”) Master's Thesis, Universidad Santo Tomás, Bogotá, 2022
- , Methodology for the Calculation of Climate Comfort in Colombia”, (In Spanish, Metodología para el Cálculo del Confort Climático en Colombia”), Institute of Hydrology, Meteorology and Environmental Studies - IDEAM, 19982024
Predictive value of three thermal comfort indices in low temperatures on cardiovascular morbidity in the Iberian peninsula ,Science of The Total Environment , Vol. 729 ,pp 138969 , 2020, https://doi.org/https://doi.org/10.1016/j.scitotenv.2020.138969
, Thermal Comfort and the Heat Stress Indices ,Ind Health , Vol. 44 (3),pp 388–398 , 2006, https://doi.org/https://doi.org/10.2486/indhealth.44.388
, La confortabilidad climática en los parques Las Peñas-Los Ocotillos y sus efectos en el bienestar humano / The climatic comfort in Las Peñas – Los Ocotillos parks and its effects on human welfare ,CIBA Revista Iberoamericana de las Ciencias Biológicas y Agropecuarias , Vol. 4 (8),pp 118 , 2016, https://doi.org/https://doi.org/10.23913/ciba.v4i8.37
, Climate information for building designers: a graphical approach ,Archit Sci Rev , Vol. 61 (1–2),pp 58–67 , 2018, https://doi.org/https://doi.org/10.1080/00038628.2017.1389690
, Evaluation of innovative sustainable design techniques from traditional architecture: a case study for the cold dry climatic region in Turkey ,Archit Sci Rev , Vol. 61 (3),pp 143–155 , 2018, https://doi.org/https://doi.org/10.1080/00038628.2018.1457509
, Modeling Off-Shore Wind Turbine Construction Project Subject to Impact of Wind Uncertainty ,World Journal of Engineering and Technology , Vol. 07 (02),pp 38–47 , 2019, https://doi.org/https://doi.org/10.4236/wjet.2019.72B005
, Skin Blood Flow in Adult Human Thermoregulation: How It Works, When It Does Not, and Why ,Mayo Clin Proc , Vol. 78 (5),pp 603–612 , 2003, https://doi.org/https://doi.org/10.4065/78.5.603
, A Tutorial on Expository Discourse: Structure, Development, and Disorders in Children and Adolescents ,Am J Speech Lang Pathol , Vol. 25 (3),pp 306–320 , 2016, https://doi.org/https://doi.org/10.1044/2016_AJSLP-14-0130
, Open windows for natural airflow and environmental noise reduction ,Archit Sci Rev , Vol. 61 (5),pp 338–348 , 2018, https://doi.org/https://doi.org/10.1080/00038628.2018.1502151
, ASHRAE 55 adaptive model application in hot and humid climates: the Brazilian case ,Archit Sci Rev , Vol. 58 (1),pp 93–101 , 2015, https://doi.org/https://doi.org/10.1080/00038628.2014.981145
, - , Building Energy Efficiency Standards for Residential and Nonresidential Buildings, 20192024